Air transportation safety investigation report A19O0089
Loss of control and collision with terrain
Hawk Air (705833 Ontario Ltd.)
de Havilland DHC-2 Mk. I (Beaver), C-FBBG
Hawk Junction Water Aerodrome, Ontario
The Transportation Safety Board of Canada (TSB) investigated this occurrence for the purpose of advancing transportation safety. It is not the function of the Board to assign fault or determine civil or criminal liability. This report is not created for use in the context of legal, disciplinary or other proceedings. See Ownership and use of content.
-
Table of contents
Summary
On 11 July 2019, at approximately 0852 Eastern Daylight Time, the float-equipped de Havilland DHC-2 Mk. I Beaver aircraft (registration C-FBBG, serial number 358), operated by Hawk Air, departed from the Hawk Junction Water Aerodrome, on Hawk Lake, Ontario. The aircraft, with the pilot and 1 passenger on board, was on a daytime visual flight rules charter flight. The aircraft was going to drop off supplies at an outpost camp on Oba Lake, Ontario, approximately 35 nautical miles north-northeast of the Hawk Junction Water Aerodrome.
The aircraft departed heading northeast. Shortly after takeoff, during the initial climb out, just past the northeast end of Hawk Lake, the aircraft crashed in a steep nose-down attitude, severing a power line immediately before impact, and coming to rest next to a hydro substation.
The pilot and the passenger received fatal injuries. The aircraft was destroyed as a result of the impact, but there was no post-impact fire. The emergency locator transmitter activated on impact, and the signal was received by the Joint Rescue Coordination Centre in Trenton, Ontario.
1.0 Factual information
1.1 History of the flight
On 11 July 2019, at 0700,Footnote 1 the pilot of the float-equipped de Havilland DHC-2 Mk. I Beaver aircraft (registration C-FBBG, serial number 358), operated by Hawk Air, began his duty day in anticipation of an 0800 flight departure from the Hawk Junction Water Aerodrome (CNH6), on Hawk Lake, Ontario. He conducted pre-flight preparations and fuelled the aircraft for the charter flight to drop off goods and supplies at a remote camp on Oba Lake, Ontario, approximately 35 nautical miles (NM) north-northeast of CNH6. He loaded the cargo with the assistance of a camp maintenance worker who was employed by Hawk Air and was flying as a passenger. Due to low overcast cloud, departure was delayed until the weather became suitable for flight under visual flight rules (VFR). At approximately 0840, the pilot and the passenger boarded the aircraft and the pilot started the engine. The takeoff direction was toward the northeast, which required taxiing for approximately 10 minutes toward the southwest end of the lake for departure.
At approximately 0852, the aircraft began its take-off run. It became airborne approximately abeam the Hawk Air dock (Figure 1), and climbed to an estimated height of 300 to 400 feet above ground level (AGL). The aircraft was observed to be climbing normally before entering a sudden left bank and an extreme nose-down attitude.
In the vicinity of the accident site, the sound of the engine was abruptly diminished, as if the engine was suddenly operating at a low engine power setting or was not running. The sound of an aircraft impacting the ground was heard shortly after.
At 0853, the aircraft collided with terrain beside a hydro substation, just outside the town of Hawk Junction. The pilot and passenger were fatally injured. The aircraft was destroyed, but despite a significant amount of fuel leaking, there was no post-impact fire. The emergency locator transmitter (ELT) activated on impact, and the signal was received by the Joint Rescue Coordination Centre in Trenton (Ontario).
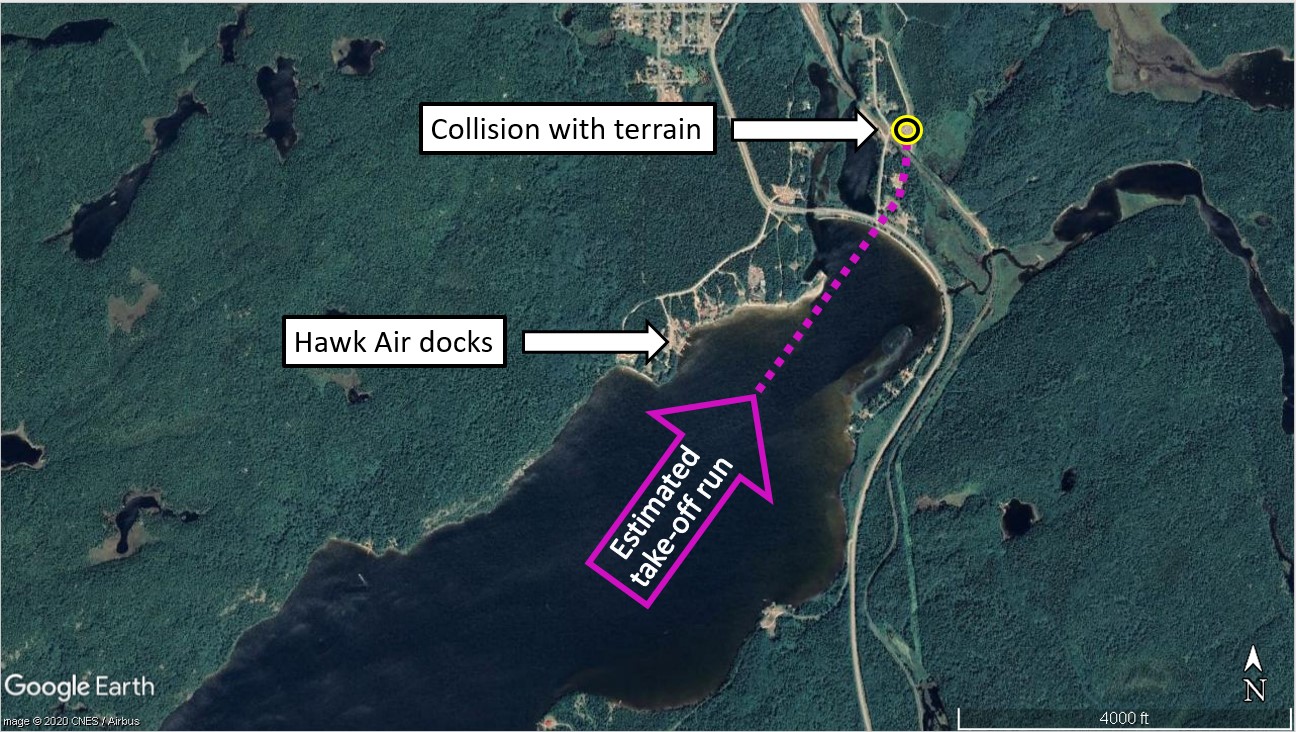
1.2 Injuries to persons
Injuries | Crew | Passengers | Total in the aircraft |
---|---|---|---|
Fatal | 1 | 1 | 2 |
Serious | 0 | 0 | 0 |
Minor | 0 | 0 | 0 |
None | 0 | 0 | 0 |
Total | 1 | 1 | 2 |
1.3 Damage to aircraft
The aircraft was destroyed as a result of the collision with terrain.
1.4 Other damage
One hydro line was severed by the aircraft’s left elevator immediately before the collision with terrain. There was minor damage to the chain link fence enclosing the hydro substation adjacent to the collision site. Hydro service was interrupted to nearby communities for approximately 2 hours.
1.5 Personnel information
The pilot was certified and qualified for the flight in accordance with existing regulations.
The pilot began his employment at Hawk Air in May 2016, at the start of the 2016 float flying season. He completed company training and began flying the company’s Cessna 180. Later in the 2016 season, he began to receive training in the occurrence aircraft and continued his training during the 2017 season. In the 2018 and 2019 seasons, he was the primary DHC-2 pilot at Hawk Air.
Pilot | |
---|---|
Pilot licence | Commercial pilot licence - aeroplane |
Medical expiry date | 01 November 2019 |
Total flying hours | 1231.2 |
Flight hours on type | 409.5 |
Flight hours in the last 7 days | 18.2 |
Flight hours in the last 30 days | 68.3 |
Flight hours in the last 90 days | 133.6 |
Flight hours on type in the last 90 days | 122.7 |
Hours on duty before the occurrence | 1.9 |
Hours off duty before the work period | 40 |
The occurrence pilot worked 6 days a week, Thursday to Tuesday, and had Wednesday off. The occurrence took place during his first flight on a Thursday, following his scheduled day off.
1.6 Aircraft information
Manufacturer | de Havilland Aircraft of Canada Ltd. |
---|---|
Type, model and registration | DHC-2 Mk. I |
Serial number | 358 |
Certificate of airworthiness/flight permit issue date | 02 May 1988 |
Total airframe time | 17 804.2 hours |
Engine type (number of engines) | Pratt & Whitney R-985-AN-14B radial, 9 cylinders, air-cooled (1) |
Propeller/Rotor type (number of propellers) | Hamilton Standard 2D30-237 (1) |
Maximum allowable take-off weight | 5090 lbs (2308.79 kg) |
Recommended fuel type(s) | 100LL |
Fuel type used | 100LL |
The most recent maintenance on the occurrence aircraft had taken place on 17 June 2019, when the aircraft underwent a 100-hour periodic inspection. At the time of the occurrence, the aircraft had accumulated 36.4 hours since this inspection.
The engine had accumulated 1013.7 hours since overhaul. Hawk Air’s approved maintenance schedule for the DHC-2 requires the engine to be overhauled at intervals of 1400 hours.
The investigation revealed nothing that would indicate that any airframe, flight control, or engine malfunctions contributed to this occurrence.
Records indicate that the aircraft was certified, equipped, and maintained in accordance with existing regulations and approved procedures.
1.6.1 Weight and balance
The occurrence aircraft’s maximum permissible take-off weight was 5090 pounds. The load record for the occurrence flight was found at the site of the accident, and indicated a take-off weight of 5010 pounds. Weighing of the cargo items found at the occurrence site suggests that the cargo weight was accurately recorded on the load record.
The investigation was unable to confirm how much fuel had leaked from the aircraft following the occurrence. The load record for the occurrence flight indicated a fuel load of 210 pounds (approximately 29 imperial gallons).
1.6.2 Fuel system
The DHC-2 Mk. I aircraft contains 3 fuel tanks located beneath the cabin floor and designated front, centre, and rear tanks. Fuel is added through individual filler necks located in a compartment with a hinged door on the left side of the fuselage, adjacent to the cockpit door.Footnote 2 The front and centre tanks each have a capacity of 29 imperial gallons, while the rear tank has a capacity of 21 imperial gallons. The occurrence aircraft was also equipped with wingtip fuel tanks; however, these were not being used by Hawk Air, and they were appropriately placarded.
During normal engine operation, fuel pressure is provided by an engine-driven fuel pump. A wobble pump is used to build up fuel pressure before engine start, or to maintain fuel pressure should the engine-driven fuel pump fail.
1.6.2.1 Fuel selector
To select a fuel tank in the DHC-2 Mk. I aircraft, the pilot operates a 4-position D-shaped handle selector located on the lower left side of the instrument panel in the cockpit. The handle is shaped in such a way as to function as a pointer, with a raised arrow on the top of the handle, which points to the selected tank (Figure 2).
The fuel selector positions available are OFF, FRONT TANK, CENTRE TANK, and REAR TANK. The selector cannot be turned clockwise from the REAR TANK position to the OFF position, nor can it be turned counter-clockwise from the OFF position to the REAR TANK position.
The fuel selector is connected with cables to the cable-actuated selector valve located in the belly of the aircraft, aft of the rear fuel tank.
The fuel selector was found to be set to the REAR TANK position at the occurrence site, as shown in Figure 2. The cable-actuated selector valve was also found to be positioned for the rear fuel tank to be the fuel source.
1.6.2.2 Fuel pressure
The DHC-2 Mk. I aircraft is equipped with a fuel pressure indicator located on the engine instrument panel. It is also equipped with a red low fuel-pressure warning light that is positioned above the flight instrument panel and that illuminates whenever the fuel pressure drops below 3 psi.
An examination of the low fuel-pressure warning light by the TSB laboratory indicated that the light was illuminated at the moment of impact.
1.6.2.3 Carburetor icing
Any carbureted aircraft engine is susceptible to carburetor icing under certain atmospheric conditions: high relative humidity (above 80%) and outside air temperatures as high as 20 °C. On the day of the occurrence, the air temperature was 14 °C, while the dew point was 13 °C, which creates the potential for serious carburetor icing.Footnote 3 Ice can form inside the carburetor as intake air is cooled by the venturi effect, restricting the flow of air and fuel to the engine. Power loss will result, and if the signs go undetected, a total loss of power can occur. Aircraft use a carburetor heat control to introduce warm air into the carburetor in order to either keep ice from forming or to melt any ice that has already formed. Carburetor heat is not normally used during takeoff because it diminishes engine performance.
1.6.3 Stall characteristics
According to the DHC-2 flight manual, the “stall is gentle at all normal conditions of load and flap and may be anticipated by a slight vibration, which increases as flap is lowered.” However, during a stall, “[i]f yaw is permitted, the aircraft has a tendency to roll.” The pilot must immediately take corrective action to prevent the roll from developing.Footnote 4 The manual also states “[i]n tight turns, flight load factors may reach the limit loads, and may also increase the danger of an unintentional stall.”Footnote 5
1.6.4 Stall warning system
Aircraft design standardsFootnote 6 require that aircraft certified in the normal, utility, aerobatic, and commuter categories be equipped to provide the pilot with a clear and distinctive stall warning, with the flaps and landing gear in any normal position, in straight and in turning flight. The standards also state that:
[t]he stall warning may be furnished either through the inherent aerodynamic qualities of the aeroplane or by a device that will give clearly distinguishable indications under expected conditions of flight. However, a visual stall warning device that requires the attention of the crew within the cockpit is not acceptable by itself.Footnote 7
Flight tests completed during certification of the DHC-2 type in the 1940s determined that the aerodynamic buffeting near the stall was a clear and distinctive stall warning. As this was deemed to have met the design requirements, no further device or stall warning systemFootnote 8 was mandated to be installed.
In practice, very few aircraft types still in commercial operation today were type-certified without a stall warning system. The few types remaining in commercial operation, including the DHC-2, were certified before 1960.
Since 1998, the TSB has investigated 14 occurrences (not including this one) involving a de Havilland DHC-2 stalling and crashing (Appendix A), resulting in 38 fatalities.
The occurrence aircraft was not equipped with a stall warning system, nor was it required to be by regulations.
1.6.4.1 DHC-2 stall warning system modification
Although the occurrence aircraft was not originally equipped with a stall warning system, such a system is available for the DHC-2, in the form of an approved modification (MOD 2/973) from Viking Air Limited, which is the current holder of the DHC-2 type certificate. Viking Air Limited has also designed an improved modification (MOD 2/1605) to the previously offered stall warning system that provides a visual and aural warning of an impending stall.
In late June 2014, Viking Air Limited published a technical bulletin recommending that stall warning systems be installed or enhanced on all DHC-2s via MOD 2/1605.Footnote 9 In addition, Transport Canada (TC) published a Civil Aviation Safety Alert in 2014 in which it also recommended that all DHC-2 airplane owners incorporate MOD 2/1605 or another approved artificial stall warning system.Footnote 10
1.6.4.2 Previous TSB recommendation on DHC-2 stall warning systems
In October 2013, at the conclusion of TSB air transportation safety investigation A12O0071, the Board issued a safety concern that the aerodynamic buffet of DHC-2 aircraft alone may provide insufficient warning of an impending stall. The TSB noted the high frequency of accidents caused by an aerodynamic stall, as well as the catastrophic consequences of these accidents when they occur at low altitude and during critical phases of flight.
In August 2017, along with the release of TSB Aviation Investigation Report A15Q0120, involving a DHC-2 Mk. I aircraft that stalled during a low-altitude turn while on a sightseeing flight, the Board recommended that
the Department of Transport require all commercially operated DHC‑2 aircraft in Canada to be equipped with a stall warning system.
TSB Recommendation A17-01
In its March 2019 response, TC had committed to undertake an in-depth study to determine the most effective means of addressing the risks associated with stall-related accidents in DHC-2 aircraft. In its December 2019 update, TC advised that it had completed the study and concluded that it would not require all commercially operated DHC-2 aircraft in Canada to be equipped with a stall warning system.
The TSB does not agree with TC’s statement that “even with a stall warning system installed, a stall occurs and gives the pilot little to no time to react and recover.”
Since no new measures will be taken by TC to address the risks associated with stall-related accidents in DHC-2 aircraft, the Board believes that the risks associated with the safety deficiency identified in Recommendation A17-01 remain.
Therefore, the response to Recommendation A17-01 was assessed as Unsatisfactory.Footnote 11
1.6.5 Normal takeoff
The DHC-2 flight manual indicates that a normal takeoff is made with the fuel selector at the desired position, flaps in the TAKE-OFF position,Footnote 12 and at maximum permissible take-off power.Footnote 13 At a safe height, a power reduction is required, and a climb speed of 80 mph should be established, which is the speed for the best angle of climb.Footnote 14 According to the flight manual, the flaps should be retracted to the CLIMB setting at an altitude of 500 feet.Footnote 15
Many DHC-2 operators, including Hawk Air, choose to retract flaps to the CLIMB setting following the initial power reduction when obstacle clearance is assured, which would result in the flaps being set to CLIMB at an altitude lower than 500 feet.
1.6.6 Emergency procedures
1.6.6.1 Engine failure after takeoff
The DHC-2 flight manual contains a section on engine failures after takeoff: the first item calls for the pilot to “[l]ower the nose immediately, to maintain airspeed at 65 mph.”Footnote 16,Footnote 17 The final item of the procedure is capitalized and is followed by 2 caution messages:
(j) KEEP STRAIGHT AHEAD AND CHANGE DIRECTION ONLY ENOUGH TO MISS OBSTACLES. USE RUDDER ONLY.
CAUTION
Always maintain enough airspeed to assure full control of aircraft to point of touchdown. Coarse use of ailerons near the stall airspeed precipitates wing dropping.
CAUTION
It is better to ride an aircraft with a dead engine safely to a crash landing straight ahead, than to turn back to the field. Attempts to turn back have, in many instances, ended with an uncontrolled roll or spin into the ground.Footnote 18
The DHC-2 flight manual also contains guidance for an engine failure above 800 feet after takeoff, requiring a glide speed of 92 mph, and allowing for a decision to turn back to the departure point if altitude allows.
A gliding distance chart is included in the flight manual. A note below the chart indicates that a float-equipped DHC-2 with flaps up, gliding at 92 mph in still air, will cover a straight line distance of 3¼ statute miles for every 2000 feet of altitude above ground.Footnote 19 The gliding distance chart does not include data for DHC-2 gliding distances at 65 mph.
1.6.7 DHC-2 aircraft flight controls
The primary flight controls of the DHC-2 aircraft are conventional and consist of a control column and rudder pedals. The occurrence aircraft did not have a handwheel on the right side (that of the co-pilot). However, it did have a control column, the upper portion of which includes the handwheel, and can tilt from left to right. It is held in position by a bolt in the hinge (Figure 3). The flight manual describes the procedure used to move the control wheel from side to side:
A lock plunger at the hinge point of the control column locks the hinged upper portion of the column in position.
The control column can be thrown over during level cruising flight without disturbing the balance of the aircraft by grasping the upper portion of the column and allowing the handwheel free movement as the upper portion is “thrown over” for use by the co-pilot.Footnote 20

1.7 Meteorological information
The nearest reporting station to the occurrence site was at Wawa Airport (CYXZ), Ontario, approximately 11 NM southwest of CNH6. At the time of the occurrence,
- the wind was variable between 310° true (T) and 010°T at 12 knots and gusting to 18 knots,
- visibility was 15 statute miles,
- the temperature was 14 °C and dew point was 13 °C,
- the ceiling was broken at 1200 feet AGL, and there were additional broken layers of cloud at 2400 feet AGL and 4200 feet AGL.
1.8 Aids to navigation
Not applicable.
1.9 Communications
Not applicable.
1.10 Aerodrome information
CNH6 is a registered private water aerodrome located on Hawk Lake, approximately 1 NM south of the town of Hawk Junction, at an elevation of 1030 feet mean sea level.
Hawk Lake is oriented on a southwest to northeast axis, and is over 10 000 feet long. The lake is over 2000 feet wide at its midpoint, narrowing to approximately 900 feet at the northeast end.
Prevailing winds are from the west or southwest in the summer, resulting in most of the takeoffs being conducted toward the southwest, originating near the Hawk Air dock.
The geography surrounding CNH6 includes heavily forested areas, areas of rising terrain, small streams, marshlands, and lakes. Aside from a water landing, there are very few places on land where a forced landing could be conducted without the likelihood of significant damage to the aircraft and potential injury to the occupants.
1.11 Flight recorders
The aircraft was not equipped with a flight data recorder or a cockpit voice recorder, nor was either required by regulations.
1.12 Wreckage and impact information
At the site of the accident, a hydro pole located near the trailing edge of the left wing and a fence located near the trailing edge of the right wing were undamaged. This is consistent with the aircraft impacting the gravel-covered terrain in a very steep nose-down attitude; the left wing was slightly lower than the right. The damage to the aircraft was consistent with the early stages of an incipient spin.Footnote 21 A single electrical transmission line (of a set of 3 transmission lines) was severed by the left elevator, which became separated from the aircraft as a result.
The propeller blades showed chordwise scratching, and one blade had dug into the gravel surface. However, damage to the propeller hub suggests very little rotation at the time of impact. There was significant impact damage to the engine case and cylinders, and to the accessory parts on the rear of the engine.
The fuselage ahead of the rear float strut attachment points had significant compression damage, and the livable space in the cockpit was compromised.
The front and centre fuel tanks were ruptured, and fuel leaked from the aircraft soon after the impact. The rear fuel tank was undamaged, and did not contain any traces of fuel. Examination of the fuel system at the site did not reveal the presence of fuel in the selector valve itself, the fuel lines leading to the engine, or the carburetor float bowl. The fuel system downstream of the selector valve, up to and including the carburetor, was damaged by the impact, which allowed fuel to drain from the wreckage.
A detailed examination of the engine and its accessory parts did not reveal any mechanical anomalies that may have existed before the impact.
The flap actuator was recovered at the site, and measurement of the actuator position indicated that the flaps were set to CLIMB at the time of the occurrence.
Many of the flight instruments and engine gauges were significantly damaged as a result of the impact; the portions that were recovered were sent to the TSB Engineering Laboratory in Ottawa, Ontario, for analysis. Due to impact damage, there was very little information recovered during this analysis; however, the information that was recovered was consistent with the other information gathered by the investigation.
A largely intact Garmin Aera 500 device was found at the site; however, an examination by the TSB laboratory of the non-volatile memory contained on the device found that the device was not configured to record flight data and it did not provide any information about the occurrence flight.
Portions of a JPI engine monitoring device were also recovered from the wreckage; however, the analysis of the non-volatile memory contained on the device did not provide any information about the occurrence flight.
1.13 Medical and pathological information
The investigation determined that there was nothing to indicate that the pilot's performance was degraded by medical or physiological factors.
1.14 Fire
Not applicable.
1.15 Survival aspects
The aircraft was fitted with an automatic fixed ELT (Artex ME-406) transmitting on 406 MHz and 121.5 MHz. It had been installed just behind the baggage compartment on the right side of the fuselage. It activated as designed following the collision with terrain. The operator received a phone call from the Joint Rescue Coordination Centre as a response to the ELT activation, shortly after the occurrence.
Not long after, the Ontario Provincial Police was dispatched to assess and secure the site.
The occupants were found in the aircraft. The livable space within the cockpit had been reduced significantly as it was crushed between the engine and the cargo that shifted forward at impact. Both front seats were badly deformed, indicating high vertical forces at impact. The accident was not survivable due to those forces.
1.15.1 Safety belts
The occupants were found with their respective lap straps attached and buckled. They were not wearing their shoulder harnesses at the time of the occurrence, although this would not have affected the survivability in this occurrence.
1.15.1.1 Regulatory requirements
The Canadian Aviation Regulations (CARs) contain several requirements for aircraft to be equipped with safety belts, restraint systems, and shoulder harnesses. More specifically, the CARs stipulate the following with respect to the requirement for seats and safety belts:
605.25 (1) The pilot-in-command of an aircraft shall direct all of the persons on board the aircraft to fasten safety belts
- during movement of the aircraft on the surface;
- during take-off and landing; and
- at any time during flight that the pilot-in-command considers it necessary that safety belts be fastened.Footnote 22
A safety belt is defined in the CARs as “a personal restraint system consisting of either [emphasis added] a lap strap or a lap strap combined with a shoulder harness.”Footnote 23 The CARs define a shoulder harness as “any device that is used to restrain the upper torso of a person and that consists of a single diagonal upper torso strap or dual upper torso straps.”Footnote 24
While the intent of this regulation is that all available parts of the safety belt system should be used, the regulation can be interpreted to mean that wearing the lap strap only would be compliant.
The investigation found that the shoulder harnesses available in the DHC-2 and other Hawk Air aircraft were not commonly used by its pilots, and they were not aware that the intent of the CARs was to require the use of a shoulder harness (when it is available) with the lap strap.
1.15.1.2 Previous TSB recommendation on the definition of safety belt
The use of a 3- or 4-point restraint system (lap strap and shoulder harness) ensures a more equal distribution of the impact forces and reduces the severity of injuries to the upper body and head.
The TSB has investigated many accidentsFootnote 25 involving aircraft that were equipped with detachable shoulder harnesses where it was determined that the harnesses were not being worn at the time of the accident.
Following a helicopter accident at Tweed, Ontario,Footnote 26 the TSB investigation determined that the passengers’ shoulder harnesses were not used with the lap straps. While TC has published various documents in an attempt to clarify the definition of “safety belt” in the regulations, if regulations are not clear in requiring the use of all available components of a safety belt, shoulder harnesses may not be used as intended, increasing the risk of injury or death. Therefore, the Board recommended that
the Department of Transport amend the Canadian Aviation Regulations to remove any ambiguity associated with the definition of “safety belt.”
TSB Recommendation A19-01
In its January 2020 response, TC indicated that it agrees with Recommendation A19-01 and that it has begun assessing the regulatory impact of changing the definition of “safety belt” in subsection 101.1(1) of the CARs. TC has also published guidance material concerning the correct use of safety belts.
The Board is encouraged that TC has initiated work to address this safety deficiency. A change in the definition of “safety belt,” when fully implemented, will mitigate the risk associated with the safety deficiency identified in Recommendation A19-01.
Therefore, the response to Recommendation A19-01 is assessed as Satisfactory Intent.
1.16 Tests and research
1.16.1 TSB laboratory reports
The TSB completed the following laboratory reports in support of this investigation:
- LP157/2019 – NVM Data Recovery
- LP158/2019 – Flight Instrument and Gauges Analysis
- LP159/2019 – Fuel Selector Analysis
- LP178/2019 – Fuel Pressure Warning Lamp Analysis
- LP236/2019 – Engine Examination
1.17 Organizational and management information
1.17.1 Hawk Air
Hawk Air is a family-run business that has been operating out of CNH6 for over 30 years. Hawk Air is a TC-approved commercial air operator conducting flight operations under Subpart 703 (Air Taxi Operations) of theCARs. Hawk Air’s flight operations are seasonal between May and October, and all of the operator’s aircraft are float-equipped. At the time of the occurrence, Hawk Air’s fleet consisted of 3 aircraft: 1 Cessna 180 (C-180), 1 de Havilland Otter with a turbine-engine conversion (DHC-3T), and 1 de Havilland Beaver (DHC-2 Mk. I), which was the occurrence aircraft. All of the aircraft were based at CNH6, where Hawk Air is the sole operator.
The primary business of Hawk Air is fly-in vacations, mostly for the purpose of fishing. The company operates a network of remote camps and its aircraft are used to transport passengers and camp maintenance personnel, as well as cargo, to these camps. Hawk Air also conducts charter flights carrying passengers and/or cargo to camps owned privately or by other companies. At the time of the occurrence, it employed 3 full-time pilots, 2 of whom also occupied management positions at Hawk Air.
1.17.2 Air-taxi training
The CARs require air-taxi operators to “establish and maintain a ground and flight training program.”Footnote 27 Section 723.98 of the Commercial Air Service Standards (CASS) specifies that “[t]he syllabus of each training program shall include the programmed time allotted and subject matter to be covered.”Footnote 28 Initial training for the DHC-2 requires 5.5 hours of ground training and 3 hours of in-flight training,Footnote 29 while annual recurrent training requires 2.5 hours of ground training and 1 hour of in-flight training.Footnote 30
Subsection 723.88(2) of the CASS states that for pilots flying day VFR only, as is the case at Hawk Air, “the chief pilot, or a pilot delegated by the Chief Pilot, shall be responsible for the training and shall certify the competency of each pilot on the most complex single-engine aeroplane to be flown.”Footnote 31 This certification is known as a pilot competency check and is completed on an annual basis in conjunction with recurrent training.
The air-taxi sector includes a wide variety of operators, operating environments, and aircraft types, configurations, and classes. The training requirements for airborne training required by TC for this diverse sector do not include many items that are specific to a particular type or class of aircraft. Individual operators are left to determine how to address the training that may be required for their specific aircraft types and classes, and for their type of operation. An operator’s training program is outlined in its operations manual, which is approved by TC. The approved training program is considered to be adequate as long as the training is provided to the pilots as set out in the manual. To assess compliance and ensure that all applicable training has been completed, TC can verify the completed training forms.
1.17.2.1 Airborne training
Many air-taxi operators in Canada use aircraft for which there is no flight simulator that can replicate aircraft performance in realistic conditions, especially in a floatplane configuration. As a result, the training must take place while in flight.
Subsection 723.98(10) of the CASS, which sets out the requirements for airborne training programs, begins with the following statement: “Any simulated failures of aeroplane systems shall only take place under operating conditions which do not jeopardize safety of flight.”Footnote 32
Three of the exercises required by the CASS pertain to this occurrence:
(a) Standard Operating Procedures for normal, abnormal and emergency operation of the aeroplane systems and components including: [...]
(vi) simulated engine fire and failure;
[...]
(xvii) approach to the stall and recovery procedure simulating ground contact imminent and ground contact not a factor (clean, take-off and landing configuration);
(xviii) buffet onset boundary, steep turns (45° of bank) and other flight characteristics (as applicable for initial and upgrade only)[…]Footnote 33
The airborne training requirements for air-taxi operators stipulate that an approach to stall must be made, with clean, take-off, and landing flap configurations. It is also required to simulate one of these stalls with what CASS terms “ground contact imminent,” which is done by assigning an altitude that represents the ground level.Footnote 34 There is no requirement for the aircraft to be fully stalled during airborne training.
TC does not provide any guidance on how these manoeuvres are to be demonstrated by a training pilot or performed by the pilot being trained, either during initial training or recurrent training. Operators can find specific guidance for many of the training manoeuvres in the applicable aircraft flight manual. Generic guidance can be found in TC’s Flight Instructor Guide (TP 975).
1.17.2.2 TSB air transportation safety issue investigation report on air-taxi operations in Canada
On 07 November 2019, the TSB published its air transportation safety issue investigation report on air-taxi safety in Canada.Footnote 35
One of the safety themes examined in this report is the training of pilots and other flight operations personnel.
Because of the nature and diversity of air-taxi operations, operators are exposed to risks that would not typically be seen in other types of operations (such as airline operations): unprepared landing sites, float-equipped aircraft, helicopter operations, locations with poor or no weather reporting, pilot self-dispatch, etc.Footnote 36
Many pilots entering the air-taxi sector have little experience outside of a training environment, and often a job with an air-taxi operator is their first job as a pilot. In many cases, they may also have been taught to fly by flight instructors who themselves have little or no experience in the air-taxi sector.
The industry consultations that were carried out in 2016 as part of this safety issue investigation provided information about what operators perceived to be their most significant risks, what they were doing to lessen those risks, and what more they believed needs to be done. It should be noted that this information represents the views of those who participated in the safety issue investigation, and these views have not been independently validated by the TSB. These observations also do not reflect ongoing initiatives by service providers or the regulator.
When asked which issues led to the highest risk to safety, among other topics, operators described a number of issues related to training for pilots and other flight operations personnel (e.g., flight followers or other required company positions).
Specifically, the operators perceived that:
[t]raining requirements in air-taxi operations are less stringent or have deficiencies. Training time allotted for mandatory training is too short to provide adequate training on the content, and mandatory content is being added without additional time allotted. Furthermore, training materials are unavailable or have not been modernized by Transport Canada (TC).Footnote 37
1.17.3 Hawk Air training
Hawk Air’s initial pilot training on the DHC-2 includes a minimum of 3 hours of flight time on type; the annual recurrent training includes a minimum of 1 hour of flight time on type.
Completed training is documented on company forms, which are used to track progress and verify that training has been completed.
Traditionally, the recurrent training would occur at the beginning of the season, usually in early May. However, in 2018, Hawk Air conducted the recurrent training for all 3 company pilots in October, with the rationale that the pilots would be ready to fly in May 2019 when the flying season began. However, skills can deteriorate over time, and there were several months where pilots were not flying after the recurrent training. As a result, they may not have been as skilled in emergency procedures when compared with training at the start of the season.
Training at Hawk Air was conducted by the chief pilot or the operations manager. According to the training records, the occurrence pilot had completed all required exercises satisfactorily during his recurrent annual training in October 2018.
It was common practice at Hawk Air to conduct some additional in-flight training at the start of the float flying season. This training would be conducted during positioning flights with no passengers or cargo on board, when the opportunity presented itself. During these flights, the aircraft would be at a relatively light weight with a centre of gravity closer to the forward limit than it would normally be while carrying passengers and/or cargo.
Hawk Air’s Operations Manual, approved by TC, was carried on board the occurrence aircraft. It contains policies and procedures applicable to all flight operations conducted under CARs Subpart 703 regulations and standards.Footnote 38
1.17.3.1 Flight training syllabus
Chapter 5 of the Operations Manual contains the details of the company training program. The initial flight training syllabus “includes instruction in the maneuvers [sic] and procedures listed.”Footnote 39 It is divided into 6 subsections covering 6 topics: pre-flight, takeoff, in-flight manoeuvres, landing, emergency procedures, and external load training.Footnote 40
The subsection on takeoffsFootnote 41 lists engine failure as one of the training items. Hawk Air, like all CARs Subpart 703 operators, was not required to conduct an airborne simulated engine failure after takeoff exercise during pilot training, and chose to address this training item in the form of a verbal or classroom briefing, as an airborne scenario was considered to be too risky.
Hawk Air training for engine failure during takeoff would typically consist of a ground briefing and discussion of the actions described in the DHC-2 Flight Manual, with an emphasis on landing straight ahead. These briefings did not include guidance for a minimum altitude required to initiate a turn back following engine failure after takeoff in any of their aircraft types, nor were they required to by regulation.
According to the company flight training records, the pilot had received engine failure training as part of the recurrent annual training that occurred in October 2018. In addition, it was reported that informal engine failure training was conducted during the 2019 float flying season.
The manual’s subsection on in-flight manoeuvres includes the following exercises (which are relevant to this occurrence) to be learned by the trainee:
c) medium an [sic] steep turns;
d) approach to the stall;
- clean configuration
- landing configuration
- take off configurationFootnote 42
The pilot’s training documents indicate that training for approach to the stall had also been completed in October 2018. However, the documents are not specific as to what configuration (clean, landing, or take-off) the aircraft was in during the training.
It was reported that, although not required by existing regulations, the occurrence pilot did conduct full stalls in the aircraft while in clean configuration (flaps-up) during the course of his training.
1.17.3.2 Safe training practices
Chapter 5 also contains a section on safe training practices (Table 4), which begins with this statement: “The following safe training practices shall be followed during all pilot flight training to reduce the risk of an actual accident or incident occurring.”Footnote 43
Maneuver | Restriction |
---|---|
Approach at [sic] stall | The exercise will be completed in VMC [visual meteorological conditions] conditions and at an altitude that will ensure recovery by 2000 feet AGL for the aeroplane type. |
Rejected takeoff | The exercise will be initiated at an indicated airspeed that is no greater than 50% of the take-off speed. |
Simulated engine failure | At a safe altitude for the applicable exercise and with consideration of engine operating temperatures. |
Simulated forced landing | Recovery must be completed by 200 [feet] AGL unless a suitable landing area exists. |
Simulated system failures | All malfunctions affecting aircraft control will be restored before landing. |
Stop and go or touch and go landings | Must have the required take-off distance remaining for takeoff. |
The Operations Manual contains no other guidance regarding how these exercises are to be conducted, nor is it required to do so by regulation.
1.17.4 DHC-2 fuel management
The DHC-2 flight manual contains a section on fuel management, which states: “For favourable CG [centre of gravity] travel […], [e]mpty rear tank first, if aircraft is fully loaded, in order to move the CG progressively forward.”Footnote 44
Pilots at Hawk Air were trained to fuel the front tank first, the centre tank second, and the rear tank last, taking only enough fuel for the planned flight plus VFR reserves. Pilots were also trained to empty the tanks in the reverse order: the rear tank first, the centre tank second, and the front tank last, with the fullest tank being used for takeoff and landing. Pilots at Hawk Air normally fuel their own aircraft before departure, based on their pre-flight calculations, which they enter on the load record.
For most itineraries, including that on the day of the occurrence, there would be no fuel carried in the rear tank, as the fuel required could be contained in the front and centre tanks. As the front tank was normally the fullest tank, it would normally be the one selected for takeoff and landing. The DHC-2 flight manual includes an item in the take-off checks that requires the pilot to verify or move the fuel selector position to the desired tank before commencing takeoff.Footnote 45
There is no indication that any fuel had been added to the rear tank before the departure of the occurrence flight. The aircraft had been flown the previous day by a different pilot, who had not added fuel to the rear tank. It could not be determined how much fuel was in the rear tank at the time of departure on the occurrence flight.
1.18 Additional information
1.18.1 Turning back following engine failure
If a mechanical problem occurs during takeoff that necessitates an immediate landing, pilots are faced with either attempting to carry out a forced landing in an unsuitable location—risking damage to the aircraft and injury to themselves—or attempting a 180° turn back toward the departure point.
TC’s Flight Training Manual states the following:
Numerous fatal accidents have resulted from attempting to turn back and land on the runway or aerodrome following an engine failure after take-off. As altitude is at a premium, the tendency is to try to hold the nose of the aircraft up during the turn without consideration for the airspeed and load factor. These actions may induce an abrupt spin entry. Experience and careful consideration of the following factors are essential to making a safe decision to execute a return to the aerodrome:
1. Altitude.
2. The glide ratio of the aircraft.
3. The length of the runway.
4. Wind strength/ground speed.
5. Experience of the pilot.
6. Pilot currency on type.Footnote 46
When taking off over an area that is not suitable for a forced landing, pilots benefit from having a plan for dealing with an emergency. The plan should take into account several factors, including terrain, altitude, the aircraft’s glide ratio, and wind strength. It should also include the minimum altitude at which a 180° turn would be attempted in order to return to the take-off point after an engine failure.
In 2017, the Federal Aviation Administration Safety Team published a document titled Impossible Turn,Footnote 47 in which it describes the components of a turn executed following an engine failure after takeoff, and shows how difficult this manoeuvre is to perform safely, even when flown perfectly.
1.18.2 Aerodynamic stall
An aerodynamic stall occurs when a wing’s angle of attack exceeds the critical angle at which the airflow begins to separate. When a wing stalls, the airflow breaks away from the upper surface and the amount of lift will be reduced to below that needed to keep the wing flying. While stalls occur at a given angle of attack, they can happen at any speed.
The typical recovery from a stall initially involves pushing the yoke forward (elevator down) to break the stall and achieve flying speed, levelling the wings, and applying power. When the aircraft accelerates to a speed that provides a safe margin above stalling speed, the recovery to the original or required altitude and configuration can be completed.
Airspeed is often used to predict stall conditions. The faster an airplane flies, the less angle of attack it needs to produce lift equal to weight. As the airplane slows down, the angle of attack needs to be increased to create the lift equal to weight. If an aircraft were to slow further, the angle of attack will be equal to the critical (stall) angle of attack at some point. Stall speed is the speed below which the airplane cannot create enough lift to sustain its weight in flight.
The speed at which a stall occurs depends on a number of things, including the load factor, the weight of the aircraft, and the centre of gravity.
1.18.2.1 Manoeuvring load factor
The manoeuvring load factor is “the total aerodynamic lift on the aeroplane, acting perpendicularly to the flight path, divided by the weight of the aeroplane.”Footnote 48
During straight and level flight, lift and weight are equal, and the load factor is 1. To maintain level flight when an aircraft is banked, the vertical component of lift must be increased to equal the weight of the aircraft; this is accomplished by increasing the angle of attack of the wing by pulling on the elevator control to maintain altitude (Figure 4).
![Relationship between lift and angle of bank (Source: Transport Canada, TP 1102E, Flight Training Manual: Aeroplane, 4th edition [Revised], p. 64)](./images/a19o0089-figure-04.jpg)
Increasing the angle of bank increases the load factor and the aircraft’s stalling speed because it causes the aircraft to perform as if it is heavier. At a 60° angle of bank, the load factor is 2, meaning that the aircraft performs as if it is twice as heavy as it would be in level flight. The stall speed is increased by 40% at a 60° angle of bank (Figure 5).
![Relationship between angle of bank, load factor, and basic stall speed (Source: Transport Canada, TP 1102E, Flight Training Manual Aeroplane, 4th edition [Revised], p. 63)](./images/a19o0089-figure-05.jpg)
1.18.2.2 Weight
An increase in aircraft weight results in an increase in stalling speed, as the wing is required to produce more lift to maintain level flight, bringing its angle of attack closer to the critical angle.
1.18.2.3 Centre of gravity
The location of the centre of gravity, even while remaining within aircraft limitations noted in the flight manual, will have an effect on the stalling speed and manoeuvrability of an aircraft.
A more forward centre of gravity requires more tail-down force to be applied to maintain the desired attitude; it will result in a higher angle of attack to maintain the same flight path, bringing the wing closer to the critical angle, resulting in an increased stall speed. Recovery from a stall is easier because there is less forward control input required to break the stall.
A rear centre of gravity works in the opposite manner, as it reduces the tail-down force and requires a lower angle of attack to maintain the desired flight path. This reduces the speed at which the aircraft will stall, which seems desirable; however, it has some negative effects on the stall characteristics, including decreased longitudinal stability, violent stall characteristics, and reduced control effectiveness during stall recovery.Footnote 49
1.19 Useful or effective investigation techniques
Not applicable.
2.0 Analysis
The investigation determined that the aircraft was maintained in accordance with existing rules and regulations, and that the occurrence flight was operating within the rules and guidelines laid out in the Canadian Aviation Regulations (CARs) and the company operations manual.
Therefore, this analysis will focus on the probable sequence of events that precipitated a power loss shortly after takeoff, leading to a loss of control. It will also focus on stall warning systems, pilot training, and safety belt use.
2.1 Sequence of events
The investigation revealed that a low fuel-pressure indication and power loss occurred shortly after takeoff, when the aircraft was at an altitude of approximately 300 to 400 feet above ground level. The aircraft subsequently stalled, entered a spin to the left, and impacted the ground.
Two scenarios were considered to explain why the aircraft stalled:
- Since the aircraft was near the maximum gross weight in a climb configuration when the power loss occurred, a brisk nose-down input and a significant nose-down attitude would have been required to maintain the airspeed at 65 mph. The pilot did not keep the aircraft above the stalling speed, which resulted in an aerodynamic stall leading to an incipient spin and loss of control.
- The pilot began a left turn in an effort to return to Hawk Lake, or toward a more suitable site for a forced approach. A banked attitude during a turn increases the load factor, which results in an increase in stall speed. Any tightening of the turn further increases the load factor, causing a further increase to the stall speed. Due to the aircraft’s banked attitude in a descending turn, the left wing dropped abruptly, and the aircraft entered an incipient spin. This is considered the most likely scenario.
2.1.1 Fuel starvation
Damage to the propeller indicates that there was some rotation at the time of the impact, suggesting that the propeller was windmilling. Several scenarios were considered regarding the engine power loss: carburetor icing, mechanical failure of some type, and fuel starvation. Despite the fact that carburetor icing could have caused power loss due to the ambient conditions, the investigation considered this was not likely. Mechanical failure remains a possibility, but no signs were found to support this.
During examination of the wreckage at the occurrence site, the fuel selector and cable-operated selector valve were found set to draw fuel from the rear tank, which was undamaged and contained no traces of fuel. Fuel starvation appears to be the most likely cause of the power loss experienced by the occurrence aircraft.
It could not be determined why the fuel selector was set to draw fuel from the rear tank, nor when that selection was made. These are 3 scenarios that were considered to try to explain this rear-tank selection:
- The pilot switched the fuel selector to the REAR TANK following the low fuel-pressure indication or subsequent power loss, in an attempt to re-establish the flow of fuel to the engine or possibly to select the OFF position per the emergency procedure for engine failure after takeoff. This scenario does not seem as likely as either of the other 2, as there were no mechanical deficiencies found with the engine, fuel was rarely carried in the rear tank, and the low fuel-pressure light was found to be illuminated at the time of impact.
- The fuel selector was already in the rear tank position when the pilot arrived at the aircraft on the morning of the occurrence, and he did not notice it during his pre-flight check or taxi out. It is possible that the pilot observed at a glance that the fuel selector was in the horizontal position and believed it was pointed to the front tank, like it normally was for previous flights. The REAR TANK selection is 180° from the FRONT TANK selection on the fuel selector (Figure 2); due to the pointer’s design, the opposite indication could be mistakenly verified following a casual glance by a pilot.
- The pilot became aware that there was some fuel in the rear tank and decided to be proactive by using this residual fuel during the long taxi to the take-off position. Although he intended to select the front tank before takeoff, for undetermined reasons, he did not do so.
The aircraft likely departed with the fuel selector set to the rear tank position, which did not contain sufficient fuel for departure. As a result, the engine lost power due to fuel starvation shortly after takeoff during the initial climb.
2.1.2 Turning back
The DHC-2 Beaver Flight Manual and Hawk Air’s Operations Manual both indicate that, in the event of an engine failure, the pilot should land straight ahead. In this occurrence, landing straight ahead would likely have resulted in a crash landing into a tree-covered hillside. Pilots will instinctively avoid this type of situation; however, a straight-ahead landing, even if into trees, allows the pilot to maintain control of the aircraft further into the crash sequence and improve the occupants' chances of survival. Due to the aircraft’s low altitude at the time of the power loss, the pilot would likely not have been able to glide far enough to reach a landing spot in his forward view that could reduce or eliminate the possibility of injury to himself or damage to the aircraft.
The DHC-2 Beaver Flight Manual and Hawk Air’s Operations Manual both require landing straight ahead following an engine failure after takeoff. However, after a loss of engine power at low altitude, a left turn was likely attempted in an effort to either return to the departure lake or head toward more desirable terrain for a forced landing. The aircraft stalled aerodynamically, entered an incipient spin, and subsequently crashed.
2.2 Stall warning system
The occurrence aircraft was not equipped with a stall warning system. While it may not have changed the outcome had such a system been installed, it may have given the occurrence pilot a clearer indication that a stall was imminent. Without a clear indication of imminent stall, the pilot would have had to rely on airframe buffeting during an already unfamiliar situation following a power loss.
If aircraft are not equipped with a stall warning system, pilots and passengers who travel on these aircraft will remain exposed to an elevated risk of injury or death as a result of a stall at low altitude.
2.3 Training
2.3.1 Air-taxi training requirements
The required airborne training exercises for air-taxi operators set out in Subpart 723 of the Commercial Air Service Standards (CASS) include an approach to stall, made with clean, take-off, and landing flap configurations. There is no requirement, however, to actually stall the aircraft. This prevents pilots from becoming familiar with the aircraft’s stall characteristics, and the aerodynamic cues that may occur during a developing stall.
Engine failures and forced approaches are required items in the training program, but there is no requirement to train for specific scenarios, such as an engine failure after takeoff. In fact, there are no training items specifically required for operators of single-engine or float-equipped aircraft. The concept of the turn back or the decision-making process, including establishing an altitude below which a turn back would never be attempted for the specific aircraft type, is not required to be trained. This does not, however, prevent operators from including such items in their training programs. Operators can customize their training programs based on operational requirements, as long as the programs comply with Subpart 723 of the CASS.
It would be difficult to prescribe appropriate training exercises or scenarios that would apply to all aircraft types and classes operated in the air-taxi sector. Without more comprehensive guidance from Transport Canada, the onus is on the operators to tailor their flight training to the type of operation and aircraft on which the training occurs, taking into account the associated risk factors. If air-taxi training requirements do not address the various classes of aircraft and operations included in the sector, there is a risk that significant type-, class-, or operation-specific emergency procedures will not be required to be included in training programs.
2.3.2 Hawk Air training
The investigation found that the training received by the pilot met the requirements set out in the CASS.
However, some of the Hawk Air training methods, including training during regular operations (empty or positioning flights) and briefing emergency procedures (either on the ground or while airborne) rather than demonstrating or practising them, are likely not as effective as more structured training events.
In addition, annual recurrent training was completed at the end of the 2018 operating season so that pilots would be ready for the 2019 season. Although it was not documented, it was reported that training and/or supervision did occur during the initial weeks of the 2019 float flying season. However, skills can deteriorate over time, and there were several months where pilots were not flying after the recurrent training. As a result, they may not have been as skilled in emergency procedures when compared with training at the start of the season.
If seasonal air operators conduct recurrent training at the end of the season rather than at the beginning, there is a risk that pilots will be less familiar with required emergency procedures.
Because the DHC-2 has only 1 set of controls, practising emergency procedures while airborne may result in a conservative approach by the trainer, who would be unable to take full control if the trainee were to mishandle a manoeuvre to the extent where safety was compromised. This could make manoeuvres such as an engine failure after takeoff difficult to simulate safely with a trainee at the controls.
Hawk Air deemed in-flight training for engine failures after takeoff to be a higher risk manoeuvre. Consequently, it conducted ground and/or in-flight briefings on the subject with its trainees; no actual demonstrations or in-flight training of this manoeuvre were conducted.
Hawk Air did not have a minimum turn back altitude or any discussion of the turn back manoeuvre in its training program, nor was it required to by existing guidance in the CASS.
If air operators do not tailor their airborne training programs to address emergency procedures that are relevant to their operation, there is a risk that pilots will be unprepared in a real emergency.
2.4 Safety belt use
Wearing a lap strap and a shoulder harness is known to reduce the severity of injuries, especially flailing injuries to the upper body, in the event of an accident, when compared with wearing only the lap strap. Neither the occurrence pilot nor the passenger were wearing a shoulder harness Not using the shoulder harness did not affect the survivability of this accident, however.
The investigation found that the shoulder harness available in the DHC-2 and other Hawk Air aircraft was not commonly used by its pilots, and that they were not aware that the intent of the CARs was to require the use of a shoulder harness (when it is available) with the lap strap.
If pilots and passengers do not use available shoulder harnesses, there is an increased risk of injury in the event of an accident.
3.0 Findings
3.1 Findings as to causes and contributing factors
These are conditions, acts or safety deficiencies that were found to have caused or contributed to this occurrence.
- The aircraft likely departed with the fuel selector set to the rear tank position, which did not contain sufficient fuel for departure. As a result, the engine lost power due to fuel starvation shortly after takeoff during the initial climb.
- After a loss of engine power at low altitude, a left turn was likely attempted in an effort to either return to the departure lake or head toward more desirable terrain for a forced landing. The aircraft stalled aerodynamically, entered an incipient spin, and subsequently crashed.
3.2 Findings as to risk
These are conditions, unsafe acts or safety deficiencies that were found not to be a factor in this occurrence but could have adverse consequences in future occurrences.
- If aircraft are not equipped with a stall warning system, pilots and passengers who travel on these aircraft will remain exposed to an elevated risk of injury or death as a result of a stall at low altitude.
- If air-taxi training requirements do not address the various classes of aircraft and operations included in the sector, there is a risk that significant type-, class-, or operation-specific emergency procedures will not be required to be included in training programs.
- If seasonal air operators conduct recurrent training at the end of the season rather than at the beginning, there is a risk that pilots will be less familiar with required emergency procedures.
- If air operators do not tailor their airborne training programs to address emergency procedures that are relevant to their operation, there is a risk that pilots will be unprepared in a real emergency.
- If pilots and passengers do not use available shoulder harnesses, there is an increased risk of injury in the event of an accident.
4.0 Safety action
4.1 Safety action taken
4.1.1 Hawk Air
As a result of this occurrence, Hawk Air has added more emphasis to its training for engine failures during critical phases of flight.
Hawk Air has also made it mandatory to use both the lap strap and the shoulder harness for all operations.
This report concludes the Transportation Safety Board of Canada’s investigation into this occurrence. The Board authorized the release of this report on . It was officially released on .
Appendices
Appendix A – TSB air transportation safety investigation reports of stall accidents involving DHC-2 aircraft since 1998
Occurrence | Fatalities | Synopsis |
---|---|---|
A16P0180 | 1 | The privately operated de Havilland DHC-2 on amphibious floats (registration C-GEWG, serial number 842) stalled while manoeuvring at low altitude and crashed into trees. The pilot was fatally injured, 2 of the 4 passengers received minor injuries, and the aircraft was substantially damaged. The aircraft was not equipped with a stall warning system. |
A15Q0120 | 6 | The Air Saguenay (1980) Inc. float-equipped de Havilland DHC-2 (registration C-FKRJ, serial number 210) stalled during a steep turn at low altitude and crashed into a rocky outcrop. All 6 occupants received fatal injuries. The aircraft was not equipped with a stall warning system. |
A14O0105 | 0 | The Sudbury Aviation Ltd. float-equipped de Havilland DHC-2 (registration C-FHVT, serial number 284) stalled during approach and crashed into tree-covered terrain. Two of the 3 occupants received minor injuries. The aircraft was substantially damaged. The aircraft was not equipped with a stall warning system. |
A12O0071 | 2 | The Cochrane Air Service float-equipped de Havilland DHC-2 (registration C-FGBF, serial number 168) stalled during a go-around and crashed into a lake. Two of the 3 occupants were unable to exit the aircraft and drowned. The aircraft was not equipped with a stall warning system. |
A11C0100 | 5 | The Lawrence Bay Airways Ltd. float-equipped de Havilland DHC-2 (registration C-GUJX, serial number 1132) stalled and crashed on departure. All 5 occupants received fatal injuries. The aircraft was not equipped with a stall warning system. |
A10Q0117 | 2 | The Nordair Québec 2000 Inc. de Havilland DHC-2 Mk. I amphibious floatplane (registration C-FGYK, serial number 123) stalled and crashed on departure. Two of the 5 occupants received fatal injuries. The aircraft was not equipped with a stall warning system. |
A09P0397 | 6 | The Seair Seaplanes Ltd. de Havilland DHC-2 Mk. I (serial number 1171, registration C-GTMC) stalled and crashed on departure. Six of the 8 occupants received fatal injuries. The aircraft did not have a functioning stall warning system, which the TSB noted as a cause or contributing factor. |
A08A0095 | 0 | The Labrador Air Safari (1984) Inc. float-equipped de Havilland DHC-2 (Beaver) aircraft (registration C-FPQC, serial number 873) stalled and crashed during an attempted forced landing. Five of the 7 occupants received serious injuries. The aircraft was not equipped with a stall warning system. |
A05Q0157 | 1 | The float-equipped de Havilland DHC-2 Beaver (registration C-FODG, serial number 205) stalled and crashed during departure. The pilot, who was the only occupant, received fatal injuries. The aircraft was not equipped with a stall warning system. |
A04C0098 | 4 | The Pickerel Arm Camps de Havilland DHC-2 Beaver (C-GQHT, serial number 682) stalled and crashed on approach. All 4 occupants received fatal injuries. The aircraft was not equipped with a stall warning system. |
A01Q0166 | 3 | The Air Saint-Maurice Inc. float-equipped Beaver de Havilland DHC-2 Mk. I (registration C-GPUO, serial number 810) stalled and crashed on approach. Three of the 7 occupants received fatal injuries. The aircraft was not equipped with a stall warning system, and the TSB noted this fact as a risk factor. |
A01P0194 | 5 | The Wahkash Contracting Ltd. de Havilland DHC-2 Beaver floatplane (C-GVHT, serial number 257) stalled and crashed on approach. All 5 occupants received fatal injuries. The aircraft was not equipped with a stall warning system, and the TSB noted this fact as a finding. |
A00Q0006 | 3 | The Cargair Ltd. DHC-2 Beaver (C-FIVA, serial number 515) stalled and crashed during climb. Three of the 6 occupants received fatal injuries. The aircraft was not equipped with a stall warning system. |
A98P0194 | 0 | The Air Rainbow Midcoast float-equipped de Havilland DHC-2 Beaver (C-GCZA, serial number 1667) stalled and crashed during an attempted overshoot. The occupants were not injured. The aircraft sustained significant damage. The aircraft was not equipped with a stall warning system, and the TSB noted as a cause or contributing factor the fact that the pilot had no warning of the impending stall. |